| UN UNIVERSITY LECTURES: 1 
The Evolving Gaia Theory
James Lovelock
Paper presented at the United Nations University
on 25 September 1992
Tokyo, Japan
Dr. James Lovelock is not part of a university, research institute, or business firm. He is not a wealthy man. But, as James Broughton said, "the only limits are, as always, those of vision." James Lovelock has plenty of vision. It was his foresight that created the electron capture detector, an instrument that sparked our environmental consciousness to the point where we could see pesticide residues everywhere, even in the fat of Antarctic penguins. His vision was recognized by NASA in the 1970s, when he was invited to be part of the Viking team investigating the existence of life on Mars.
The NASA experience brought Lovelock to a grand vision of our
own planet, the idea of the Earth as one big system, able to keep its temperature and composition constant in the face of change. This idea, while still controversial, is of value because it has inspired many experiments and experimenters, from scientists to politicians. It has inspired debates on the role of algae in the ocean and its control of our climate through the dimethyl sulphide mechanism. It has helped in the understanding that carbon dioxide pumped from the biota into the soil increases weathering and is a valuable sink for carbon.
During his visit to UNU, Lovelock spoke of the evolution of the Earth and of the living organisms that inhabit it, and how these led to the Gaia theory, which sees the Earth as an active self-regulating system. He also recounted the current scientific problems of biodiversity and climate change from the perspective of the Gaian view.
The notion of Gaia is not new. In a way it started about 200 years ago, in 1789, in an Edinburgh lecture given by Dr. Black, the discoverer of carbon dioxide. He gave the lecture on behalf of James Hutton, who was ill at the time. Hutton had written, "I consider the Earth to be a super-organism and that its proper study should be by physiology." James Hutton is rightly named the father of geology, but he was more than this. He was the first scientist to challenge seriously the notion that the Earth and all upon it was created intact as described in the Old Testament. He did so through his own evolutionary theory, called uniformitarianism, in which he proposed that the material environment is continuously recycled at a more or less steady state, with origins in the remote past.
James Hutton's wholesome view of our planet as a superorganism that participated in the recycling of the rocks faded away during the fierce battles between the church and science over creation or evolution. This was not from lack of evidence, but because it did not appeal as much as Darwin's great vision of the evolution of the species of organisms by natural selection. The two theories, Hutton's and Darwin's, were radical and they appeared in a cultural environment where the scriptural dogma of the act of creation was still widely held to be true. However, T.H. Huxley, Darwin's bulldog, although well aware of the significance of Hutton's theory, wisely chose to fight over the origins of the organisms, not the rocks.
Science's incompatible world views
The nineteenth century battles between science and religion succeeded in establishing Science as the prime source of knowledge. In these battles Earth and life sciences were united in their rejection of creationism. Perhaps because of this common cause, biologists and geologists never seemed to notice that their own views were insufficient when taken separately, and also contradictory. Hutton's unifying concept was lost in the fragmentation of science. As a result, two distinct and incompatible theories of evolution, Darwinism and geological evolution, became established and have existed separately from the middle of the last century until today. Let's look at the present day world views of the life and Earth scientists, and let me show you how far the Earth and life sciences have separated.
Biologists, although critical of Gaia theory, rarely comment on the Earth and the planets. Their view is normally through a microscope, not a telescope. They look at cells, genes and molecules, not planets. They are sometimes sufficiently irritated by Gaia theory to comment that the material conditions of the Earth were all explained completely by the abundance of oxygen and carbon dioxide in the air. That is how many biologists see the world today. (See Figure 1.) If they took the trouble to go back and read Huxley, they would be surprised to find he supported Hutton's view of the Earth as a superorganism.
Geochemists think otherwise. They know that the cycle of carbon dioxide between plants and animals, although large, is a "do nothing" cycle. Organisms alone do not set the level of carbon dioxide in the air, only accept what is there. Plants and animals must always, at steady state, exchange equal quantities of carbon dioxide.
Geochemists believe that their science explains it all. For example, the eminent geochemist H.D. Holland, in his book The Chemical Evolution of the Atmosphere and Oceans, said, "The regulation of the Earth's chemistry and climate can fully be described by geochemistry and geophysics alone. To geochemists there is only one source of carbon dioxide, volcanoes and only one sink for the gas, the weathering of calcium silicate rocks." (See Figure 2.)
In recent years scientists have felt uneasy about these two extreme views, and many have opted for the conglomerate science of biogeochemistry. Biogeochemistry is, in fact, a province occupied by geochemists who think that they can explain the world by including in their geochemical models boxes labeled These boxes represent living organisms as reservoirs, sources and sinks of chemicals. They are now finding that no matter how large and expensive the computers they use, nor how intricate they make these biogeochemical models, they fail to produce results that map onto the real world. Such models cannot properly account for the current low level of carbon dioxide in our atmosphere, nor the fact that oxygen remains constant at 21%. More seriously, these models, like those of population biology, are sometimes inherently unstable or prone to deterministic chaos.
There is another way of looking at the Earth scientifically; that is to go back to James Hutton's physiological view. If we take the Earth to be a superorganism, then we would not expect an expert science of a part of the system, like biology or geochemistry, to be able to explain the whole system. If the Earth does resemble a living system, then such properties as climate and chemical composition require physiology for their explanation. Try asking a biologist or biochemist how our own internal temperature is kept close to 37C. You will find them unable to answer in terms of their science. Temperature regulation can only be properly explained by physiology, the systems science of the body.
I think that to explain the regulation of the climate and the chemical composition of the Earth also requires a physiological approach. This is the justification of Gaia theory, which sees the Earth as a physiological system.
The history of the Gaia idea
This is how the modern idea of Gaia started, how it has evolved from an idea to an hypothesis, and how it has become, perhaps, a reanimation of Hutton's theory of evolution.
In 1961 I was invited to participate as an experimenter in the first NASA lunar and planetary explorations. The work involved brief visits to the famous Jet Propulsion Laboratories in Pasadena, California. I soon became interested in the methods NASA proposed to detect life on Mars. They were for the most part based on experience with laboratory organisms here on Earth. The bacteriologists proposed sending culture media to grow bacteria from the martian soil.
To me, this seemed an unsatisfactory approach. It could fail to detect the presence of life for many reasons. Martian life might not include bacteria, and even if it did, the biochemistry might be different. The experiment might land at a barren site. I suggested that they try a more general experiment, a planetary one, instead of a local search at the site of landing. For example, simply analyze the chemical composition of the martian atmosphere. If the planet were lifeless, it would be expected to have an atmosphere determined by physics and chemistry alone, and the chemical composition would be close to the chemical equilibrium state. But if the planet bore life, organisms at the surface would be obliged to use the atmosphere as a source of raw materials and as a depository for wastes. Such use of the atmosphere would change its chemical composition. It would depart from equilibrium in a way that would show the presence of life. I was joined in this study at this point by Dian Hitchcock and together we examined atmospheric evidence from the infrared astronomy of Mars. We compared this data with our knowledge of the sources and sinks of gases in the atmosphere of the one planet we knew bore life, Earth. We found an astonishing difference between the two planetary atmospheres. Mars was close to chemical equilibrium, and it was dominated by carbon dioxide. The Earth's atmosphere, in great contrast, is in a state of deep chemical disequilibrium. In our atmosphere, carbon dioxide is a mere trace gas, and the coexistence of abundant oxygen with methane and other reactive gases are conditions impossible on a lifeless planet. Even the abundant nitrogen and water are difficult to explain by geochemistry. No such anomalies are present in the atmospheres of Mars or Venus, and their existence in the Earth's atmosphere signals the presence of living organisms at the surface. Sadly, we concluded, Mars was probably lifeless.
The Earth seen from space as a dappled white and blue sphere is now a visual cliche. But when we first saw it, few of us were untouched by the beauty of our planet. That view from outside has irreversibly altered our thoughts and feelings about the Earth.
Strangely similar was the way the top-down view of atmospheric chemistry, gathered at JPL, became for me a revelation of the Earth. But this revelation was no aesthetic vision, it was hard science, the chemical analysis of the Earth's atmosphere. It revealed a gas mixture like that of the intake manifold of a car engine, oxygen and combustible gases mixed. This is very different from the exhausted, carbon dioxide dominated, atmospheres of Mars and Venus.
Much more than this, we know that the chemical composition of our atmosphere is steady and constant. Changes do occur, but only slowly compared with the residence times of the gases. One afternoon in 1965 at the JPL, when thinking of these facts, the thought came to me in a flash that such constancy required the existence of an active control system. At that time I lacked any idea of the nature of the control system, except that the organisms on the Earth's surface were part of it. I learned from astrophysicists that stars increase their heat output as they age, and that our Sun has grown in luminosity by 25% since life began. I realised that, in the long term, climate also might be actively regulated. The notion of a control system involving the whole planet and the life upon it was now firmly established in my mind. Sometime near the end of the 1960s I discussed the idea with my neighbor, the novelist William Golding. He suggested the name Gaia as the only one appropriate for so powerful an entity.
I first stated the Gaia hypothesis in 1972, in the journal Atmospheric Environment. Shortly after this I began a collaboration with the biologist Lynn Margulis that has continued to this day. The first statement of the hypothesis was: "Life regulates the climate and the chemical composition of the atmosphere at an optimum for itself."
I now realise that this statement was wrong. What we should have said is: "The whole system of life and its material environment is self-regulating at a state comfortable for the organisms." This may seem to you to be just a fine point of definition. But it laid us open to the criticism that we had proposed a sentient Gaia able to control the Earth consciously. Nothing was further from our minds. From the start, Gaia has been a top-down systems view of the Earth, the hard science view of a physical chemist with an interest in control theory. This was never some trendy new age pseudo-science.
Gaia is a proper theory that makes testable predictions. For example, here is the Gaian view of carbon dioxide. Michael Whitfield and I in a 1982 Nature paper proposed that the geochemical weathering of rocks was, by itself and in the absence of life, insufficient to account for the low contemporary level of carbon dioxide. In the real world, we observed, the system includes the organisms as well as their environment. This is a much more powerful pump for carbon dioxide than geochemistry alone, and should be able to remove carbon dioxide from the atmosphere and sustain a much cooler and, for the organisms, more comfortable climate than would otherwise be possible. We predicted that the removal of carbon dioxide from the air would depend on the growth rate of the organisms and would therefore be dependent upon the temperature. If the temperature was too low, carbon dioxide would accumulate in the atmosphere, but as the temperature rose, carbon dioxide would be removed at a faster rate, and an equilibrium temperature would be established.
In 1989 the American scientists Volk and Schwartzman confirmed part of this prediction by showing that the rate of rock weathering is increased by a factor of 1000 when organisms are present. This is much more than is needed to enable a powerful physiological regulation of climate and carbon dioxide. We think it could account for the 300-fold decline in carbon dioxide since life began on Earth.
I do not know whether Gaia theory is right or wrong. To me it is just a useful way of looking at the Earth. To illustrate what I mean by useful, I shall now describe how Gaia theory makes theoretical models easy by eliminating deterministic chaos, and conclude with a Gaian account of one of the Earth's essential systems, the sulphur cycle, and the way it may link with climate and ocean salinity.
The model Daisyworld and its lessons for biodiversity
By far the most useful and constructive criticism we received of early Gaia was from Ford Doolittle, who said that there was no way for organisms to regulate the climate other than by foresight and planning, which was impossible. I agree with him, and it was his criticism that forced me to rethink our proposal. My answer was a simple mathematical model of Gaia, called Daisyworld. This model captures the essence of what I mean by a geophysiological system.
Imagine a planet just like Earth, and orbiting a star just like the sun. This imaginary planet has a surface of bare earth, but is well watered and capable of supporting plant growth. It is seeded with daisies of two different colours, one dark and the other light. The star that warms Daisyworld is like our own sun, one that warms up as it grows older. The object of the model is to show that the simple growth and competition for space between the two daisy species can keep the temperature of Daisyworld constant and comfortable over a wide range of radiant heat output from the star.
Figure 3 shows how Daisyworld evolves. At the start of the first season, after the planetary temperature reaches 5 degrees Celsius, daisy seeds begin to germinate. After their emergence, dark coloured daisies, which absorb more heat, are at an advantage, since in the feeble sunlight they alone would be warm enough to grow. The few seeds produced at the end of the season would nearly all be of dark daisies. At the start of the next season, dark daisies would dominate and soon begin to spread, warming themselves and the area they occupied. Then, with explosive positive feedback, temperature and daisy growth would rise until a large proportion of the planetary surface was covered by dark daisies. Their growth, though, would not continue indefinitely for two reasons: first, too high a temperature suppresses growth, and second, on a warm planet there would be competition for space from light coloured, heat reflecting daisies. As the star warmed, the planetary ecosystem would change from one dominated by dark daisies to one dominated by light coloured daisies. It is the nature of stars to grow hotter as they age, and eventually the ecosystem of daisies would collapse when a total planetary surface cover of light daisies was insufficient to keep the planet cool.
The model is quite general, and works as well if the growth of the organisms alters the cloud cover or the abundance of greenhouse gases. Indeed, models of the early Earth where bacterial ecosystems regulate climate and gas composition work just as well as does Daisyworld. The geophysiological models are robust in a mathematical sense, use equations in their natural nonlinear form, and are almost entirely insensitive to the initial conditions. Daisyworld is in fact the mathematical basis of Gaia theory.
It might seem that the great stability of these geophysiological models is in contradiction to the inherent instability leading to deterministic chaos, which is a general property to evolving systems of nonlinear differential equations. It would be wrong to conclude so; Daisyworlds are more like islands of stability in a sea of chaos, the exception that "proves" the rule.
The damping effects of population density are well known to confer some stability on both natural and model ecosystems. What we have in Daisyworld is something quite different, a tightly coupled system made stable by environmental feedback. Perhaps the nearest to it is in the work of the ecologist, Tilman, who also included the environment in his models. In Daisyworld, unlimited growth does occur, but only when positive feedback is needed to bring the system rapidly to its stable state. This is illustrated in Figure 3 by the rapid rise of temperature and daisy population at the origin of Daisyworld. It may be some time before evidence and observation confirms or denies the existence of systems like Daisyworld on the Earth, although it is already possible to argue, from the geochemistry of rock weathering, that this mechanism operates for the long term regulation of atmospheric carbon dioxide and climate. For the moment let us assume it to be a fair model and see how species richness and diversity can be examined on this imaginary planet. First, there seems to be no limit to the number of species that can be accommodated in Daisyworld models. Figure 4 illustrates the evolution of climate and population on a world inhabited by thirty types of coloured plants, twelve types of herbivores, and three carnivores. During its evolution this system is perturbed continuously by a progressive growth of solar luminosity. It takes a robust system to continue to regulate and remain stable when so perturbed. The peaceful coexistence of daisies, rabbits and foxes is remarkable in itself, even without the perturbations. Note how the diversity index reaches a maximum when the system is most comfortable and is low at the extremes. This is perhaps similar to the well known increase of diversity with latitude and altitude.
Figure 5 illustrates the effect of a mild perturbation on a complex ecosystem like the one previously, when it has been allowed to run to equilibrium at a constant solar input and when the climate is comfortable. The perturbation is 4% in solar luminosity, a change similar to that between a glacial and interglacial period. Note the burst of types caused by this small change, and how the system then settles down again at its new state.
Figure 6 illustrates the relationship between the rate of application of a perturbation and the increase of the diversity index.
This analysis shows biodiversity on Daisyworld to be greatest when all is well with the ecosystem, but when rapid change, well within the limits of toleration, is taking place. Biodiversity is least when either the system is so stressed as to be near failure, or when it is healthy but there has been a prolonged period of steady state. When the system is rich in species, the relaxation time after a perturbation is very long in terms of the generation time of individual species.
Does this say anything about our present condition? We usually regard the great diversity of organisms in equatorial regions as a steady natural state. I wonder if instead we should regard this great diversity as an indication that the ecosystem or the Earth itself was healthy but has been recently perturbed. The most likely perturbation is the sudden shift from the cool period of glaciation a mere 10,000 years ago. If this view is right, then biodiversity is a symptom of change during a state of health. What seems important for sustenance is not so much biodiversity as such, but potential biodiversity, the capacity of a healthy system to respond through diversification when the need arises. In the Amazon and other regions under threat, destroying biodiversity will reduce the reservoir of apparently redundant or rare species. Among these may be those able to flourish and sustain the ecosystem when the next perturbation occurs. The loss of biodiversity rarely occurs alone; it is part of the destructive process of converting natural ecosystems to farm land. It is the whole process, the loss of biodiversity and the loss of the potential of the region to sustain biodiversity, that makes the clearance of tropical forest so dubious an act. Geophysiology also views the biodiversity of the humid tropical rain forests to be linked with their capacity to act as natural air conditioners for their region. Simple calculation shows that the value of the forests as air conditioners is worth hundreds of trillions of dollars per year.
Current worldwide Gaia research
Gaia research has been a low-key activity involving no more than about five scientists world wide, who worked on the topic in their spare time and without funding. In such circumstances it was not practical to strive hard to develop tests for the existence or otherwise of planetary scale systems. It seemed better to go into the world and collect information, inspired by the predictions from the theory.
Whether it was right or wrong seemed to matter less than that the quest was objective. A good example of this was the expedition in 1972 aboard the research ship Shackleton from the UK to Antarctica and back to seek the presence of sulphur and iodine compounds in the ocean and air. Gaia theory predicted that gaseous compounds of these elements would be dissolved in the ocean surface and present in the air above. It also predicted that these compounds would be sufficiently abundant to account for the atmospheric transport of sulphur and iodine from the sea, where they are abundant, to the land where they are scarce. This is because sulphur and iodine compounds are continuously being removed as soluble salts dissolved in stream and river water. Measurements made during the voyage found that the gases dimethyl sulphide, methyl iodide, and carbon disulphide were ubiquitous in the ocean environment. I should emphasize that the idea of the sulphur cycle including dimethyl sulphide as a major component was rejected by geochemists in the 1970s even after the voyage. It was not until the work was repeated and extensively extended by the German scientist M.O. Andreae that it was taken seriously.
It is often asked why organisms in the ocean should bother to waste energy making the volatile compound dimethyl sulphide. The most immediate answer is that they do it because the sea is too salty for them. The oceans are uncomfortably close to the upper limit of salt tolerance of mainstream life. Fish, mammals and other large ocean organisms keep their internal salinity close to 0.16 molar, the same as land animals and plants. They do this by pumping water and salts differentially. The vast surface to volume ratio of microscopic algae would make this an energetically impossible process, so they evolved a different strategy. They biosynthesize charge neutral salts whose presence within the cell balances the high osmotic pressure of the ocean without increasing the internal ionic strength to toxic levels. The most popular compound of this kind used by ocean organisms is dimethylsulphonio propionate. A single molecule with a positive charge at one end and a negative at the other. When the algae die this compound is released to the ocean where it decomposes to give acrylic acid and dimethyl sulphide. Land organisms like the vegetable beetroot are faced with a similar stress when they grow in salty places. They make a nitrogen containing charge neutral salt called betaine. In the ocean, sulphur is much more abundant than nitrogen, and organisms able to make dimethylsulphonio propionate would be at an advantage. There are many microscopic organisms in the oceans and consequently nearly all of the return of sulphur from the ocean to the land goes via dimethyl sulphide. The discovery of dimethyl sulphide as a key component of the natural environment was the first useful prediction from Gaia theory.
A global rise in temperature is a reasonable prediction as greenhouse gases, like carbon dioxide, accumulate in the air. But the extent of the temperature rise requires, among other things, an understanding of the effects and responses of clouds on climate and climate change. In a 1989 Nature paper, Mitchell suggested that the predictions based on the effects of the greenhouse gases alone may be in error by a factor of two. Mitchell's group included clouds in their general circulation model. They also took note of the effect of warming on the distribution of ice in the water of clouds. In the complex atmosphere, the solid and liquid particles floating in the air have effects far greater than their small mass would suggest.
In the early 1980s I joined with three other scientists-the German oceanographer M. O. Andreae, and the meteorologists Robert Charlson and Steven Warren. We met at the University of Washington in Seattle. We were interested in the possibility that the atmospheric oxidation products of dimethyl sulphide, sulphuric acid and methane sulphonic acid, might be the cloud condensation nuclei that otherwise appeared to be missing from the air above the oceans.
We knew that the Alaskan geophysicist, Glen Shaw, had suggested that the emission of sulphur gases from biological marine systems would provide Gaia with an economical climate regulating mechanism. His notion was that these gases would oxidise in the stratosphere and then produce an aerosol of sulphuric acid that would reflect sunlight and so cool the Earth. The process could be economical since a relatively small mass of sulphur could have a large climatic effect.
This process might have been significant at some past epoch but there was no evidence that it was playing a role in the current regulation of climate. But if sulphuric and methane sulphonic acid droplets were condensation nuclei, then there would be a huge amplification of the light reflecting tendency of these particles as they became cloud droplets. The four of us described our ideas in a Nature paper in 1987. The predictions we made linking cloud albedo to sulphur gas concentration and temperature were tested by some satellite observations by Coakley and his colleagues from NCAR later that year. They stated that our predictions for conditions over the open ocean were correct to within a few percent. It seemed as if we had discovered-through a top-down Gaia approach-a climate control mechanism as powerful as the greenhouse effect and potentially in opposition to it.
In the past five years evidence has accumulated, particularly from the careful long term investigations of Ayers and his colleagues in Australia, that establishes a direct connection between algal growth, atmospheric methane sulphonic acid and cloud condensation nuclei. This is true for the atmosphere over all the world's oceans, even the North Atlantic in spite of the huge production of cloud condensation nuclei from fossil fuel combustion on the land surfaces around it. Indeed we can now say that, but for the algal production of dimethyl sulphide (DMS), clouds over the world's oceans would be less dense and the world would be a hotter place.
An important missing link is the geophysiological connection between climate and the emission of DMS by the algae. We do not yet know if changes in the sea surface temperature affect algal growth and the algal emission of sulphur gases. A tantalizing indication of an indirect link between DMS and climate comes from observations that suggest that an increase in cloud cover, algal density and wind velocity has occurred over the oceans in the past decade.
We can speculate that the emission of DMS from an algal bloom could lead directly to a local increase in sea surface wind velocity. The energy driving the wind would come from the release of latent heat when water condensed on the cloud condensation nuclei to form the clouds. Clouds, where there was an updraught of damp air fueling this energy release, would draw in surface air and so generate wind at the sea surface. Becalmed sailors know the value of a cloud as marking a place on the ocean surface where wind can be expected. The corresponding source of biochemical energy would be the wind-induced circulation of nutrient rich water from below the thermocline. Perhaps these local systems link in with the large scale geophysical weather systems in a coherent manner and so lead to a global system response. To add speculation to supposition, I wonder if the wind increase also serves to bring nutrient elements like iron from the deserts to the global oceans.
Perhaps the most fascinating evidence about the cloud algae connection comes from ice core analyses taken in Antarctica. Data from the French scientists Delmas and Lorius and their colleagues show that as one proceeds deeper into the glacial ice above the Antarctic continent, the atmospheric carbon dioxide level in bubbles trapped in the ice remains constant until a depth corresponding to about 10,000 years ago, then it sharply declines to levels below 200 ppm and stays low throughout the last glaciation. At the same time that the carbon dioxide declined in abundance, the methane sulphonic acid level in the ice, which also had been steady during the post glacial period, suddenly doubled. This evidence suggests that during the glaciation the Earth was kept cool in part by lower carbon dioxide, and in part by denser and more reflecting clouds.
Barriers to understanding geophysiology
Many scientists including those sympathetic to Gaia theory find it difficult to understand how a system can be self-regulating when it is in positive feedback with respect to the current climate. Thus, greater warmth seems to lead to less clouds and more carbon dioxide, quite the opposite of what would be needed for self-regulation.
This paradox comes I think from our anthropocentricity; we like to think that now is normal and the ice ages abnormal. But if instead we look on the present interglacial as like a fever and the glacial state as normal, then positive feedback enables the system to return rapidly to its goal of a cooler state. It is unlikely that the full details of the cloud algae connection will be revealed for some years, yet who would deny its potential now that greenhouse warming has become a major issue?
Whether right or wrong, Gaia provides a very different, a top-down view, of our planet, in a world where science grows ever more conservative and dogmatic. I think we need to make room for the kind of errors that leads us closer to the truth. As the economist Vilfredo Pareto said, "Give me a fruitful error any time, full of seeds, bursting with its own corrections."
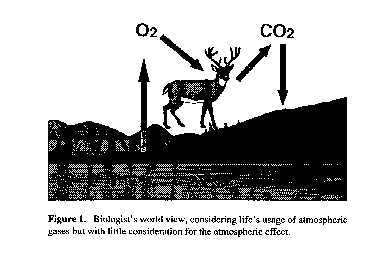
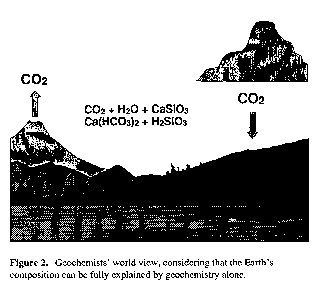
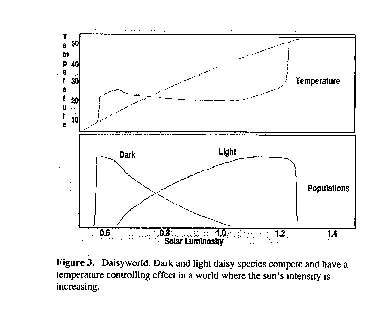


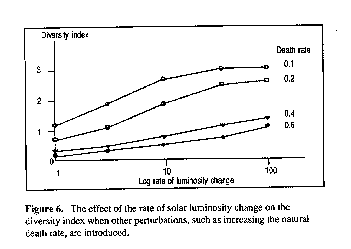
Return to previous page
Return to the UNU Homepage
|